cryopreservation of oocytes

The egg retrieval process for oocyte cryopreservation is the same as that for in vitro fertilization. This includes one to several weeks of hormone injections that stimulate ovaries to ripen multiple eggs. When the eggs are mature, final maturation induction is performed, preferably by using a GnRH agonist rather than human chorionic gonadotrophin (hCG), since it decreases the risk of ovarian hyperstimulation syndrome with no evidence of a difference in live birth rate (in contrast to fresh cycles where usage of GnRH agonist has a lower live birth rate).The eggs are subsequently removed from the body by transvaginal oocyte retrieval. The procedure is usually conducted under sedation. The eggs are immediately frozen.
The egg is the largest cell in the human body and contains a high amount of water. When the egg is frozen, the ice crystals that form can destroy the integrity of the cell. To prevent this, the egg must be dehydrated prior to freezing. This is done using cryoprotectants which replace most of the water within the cell and inhibit the formation of ice crystals.
Eggs (oocytes) are frozen using either a controlled-rate, slow-cooling method or a newer flash-freezing process known as vitrification. Vitrification is much faster but requires higher concentrations of cryoprotectants to be added. The result of vitrification is a solid glass-like cell, free of ice crystals. Indeed, freezing is a phase transition. Vitrification, as opposed to freezing, is a physical transition. Realizing this fundamental difference, vitrification concept has been developed and successfully applied in IVF treatment with the first life birth following vitrification of oocytes achieved in 1999.Vitrification eliminates ice formation inside and outside of oocytes on cooling, during cryostorage and on warming. Vitrification is associated with higher survival rates and better development compared to slow-cooling when applied to oocytes in metaphase II (MII). Vitrification has also become the method of choice for pronuclear oocytes, although prospective randomized controlled trials are still lacking.
Sperm cryopreservation
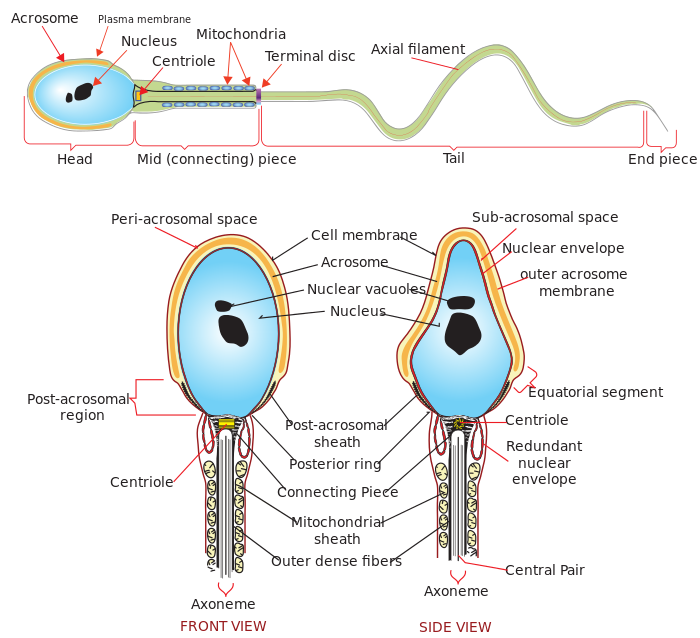
The most common cryoprotectant used for semen is glycerol (10% in culture medium). Often sucrose or other di-, trisaccharides are added to glycerol solution. Cryoprotectant media may be supplemented with either egg yolk or soy lecithin, with the two having no statistically significant differences compared to each other regarding motility, morphology, ability to bind to hyaluronate in vitro, or DNA integrity after thawing.
Additional cryoprotectants can be used to increase sperm viability and fertility rates post-freezing. Treatment of sperm with heparin binding proteins prior to cryopreservation showed decreased cryoinjury and generation of ROS.The addition of nerve growth factor as a cryoprotectant decreases sperm cell death rates and increased motility after thawing.Incorporation of cholesterol into sperm cell membranes with the use of cyclodextrins prior to freezing also increases sperm viability.
Semen is frozen using either a controlled-rate, slow-cooling method (slow programmable freezing or SPF) or a newer flash-freezing process known as vitrification. Vitrification gives superior post-thaw motility and cryosurvival than slow programmable freezing.This current technique, invented by the Japanese, is used in the best centers around the world. It is extremely fast (-23000ºC/min), so as a result it avoids the appearance of small ice crystals, preventing the "knife" effect.
Vitrification
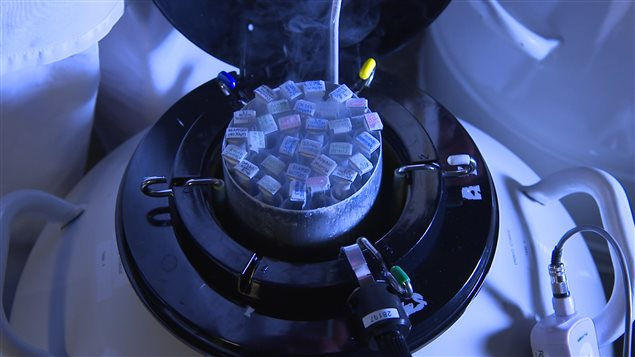
Vitrification is a flash-freezing (ultra-rapid cooling) process that helps to prevent the formation of ice crystals and helps prevent cryopreservation damage.
Researchers Greg Fahy and William F. Rall helped to introduce vitrification to reproductive cryopreservation in the mid-1980s.As of 2000, researchers claim vitrification provides the benefits of cryopreservation without damage due to ice crystal formation.The situation became more complex with the development of tissue engineering as both cells and biomaterials need to remain ice-free to preserve high cell viability and functions, integrity of constructs and structure of biomaterials. Vitrification of tissue engineered constructs was first reported by Lilia Kuleshova,who also was the first scientist to achieve vitrification of oocytes, which resulted in live birth in 1999.For clinical cryopreservation, vitrification usually requires the addition of cryoprotectants before cooling. Cryoprotectants are macromolecules added to the freezing medium to protect cells from the detrimental effects of intracellular ice crystal formation or from the solution effects, during the process of freezing and thawing. They permit a higher degree of cell survival during freezing, to lower the freezing point, to protect cell membrane from freeze-related injury. Cryoprotectants have high solubility, low toxicity at high concentrations, low molecular weight and the ability to interact with water via hydrogen bonding.
Instead of crystallizing, the syrupy solution becomes an amorphous ice—it vitrifies. Rather than a phase change from liquid to solid by crystallization, the amorphous state is like a "solid liquid", and the transformation is over a small temperature range described as the "glass transition" temperature.
Vitrification of water is promoted by rapid cooling, and can be achieved without cryoprotectants by an extremely rapid decrease of temperature (megakelvins per second). The rate that is required to attain glassy state in pure water was considered to be impossible until 2005.
Two conditions usually required to allow vitrification are an increase of viscosity and a decrease in the freezing temperature. Many solutes do both, but larger molecules generally have a larger effect, particularly on viscosity. Rapid cooling also promotes vitrification.
For established methods of cryopreservation, the solute must penetrate the cell membrane in order to achieve increased viscosity and decrease the freezing temperature inside the cell. Sugars do not readily permeate through the membrane. Those solutes that do, such as dimethyl sulfoxide, a common cryoprotectant, are often toxic in intense concentration. One of the difficult compromises of vitrifying cryopreservation concerns limiting the damage produced by the cryoprotectant itself due to cryoprotectant toxicity. Mixtures of cryoprotectants and the use of ice blockers have enabled the Twenty-First Century Medicine company to vitrify a rabbit kidney to −135 °C with their proprietary vitrification mixture. Upon rewarming, the kidney was transplanted successfully into a rabbit, with complete functionality and viability, able to sustain the rabbit indefinitely as the sole functioning kidney. In 2000, FM-2030 became the first human being to be successfully vitrified posthumously.
Two studies in the 2000s showed that the incidence of birth defects and chromosomal defects when cryopreserved oocytes were used was consistent with the incidence of natural conception.
Recent protocol modifications to cryoprotectant composition, temperature, and storage methods have greatly influenced the technique, and while it is still considered an experimental procedure, it is rapidly becoming an option for women. Traditionally, slow freezing has been the most commonly used method for cryopreserving oocytes and is the method for the largest number of babies born from frozen oocytes worldwide. Ultra-rapid freezing or vitrification represents a potential alternative to freezing.
In 2014, a Cochrane systematic review on this topic was published. It compares vitrification (the newest technique) with slow freezing (the oldest technique). The main results of this review showed that the clinical pregnancy rate in the oocyte vitrification group was almost 4 times higher than in the slow freezing group, with moderate-quality evidence.
The combination of cells
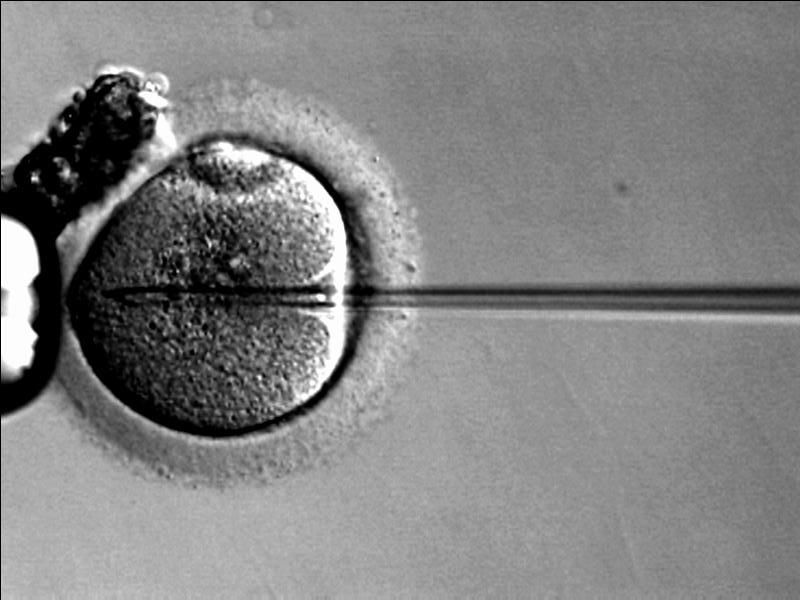
During the freezing process, the zona pellucida, or shell of the egg can be modified preventing fertilization. Thus, currently, when eggs are thawed, a special fertilization procedure is performed by an embryologist whereby sperm is injected directly into the egg with a needle rather than allowing sperm to penetrate naturally by placing it around the egg in a dish. This injection technique is called ICSI (Intracytoplasmic Sperm Injection) and is also used in IVF.
Immature oocytes have been grown until maturation in vitro, but it is not yet clinically available.
Prenatal development
Prenatal development (from Latin natalis 'relating to birth') includes the development of the embryo and of the foetus during a viviparous animal's gestation. Prenatal development starts with fertilization, in the germinal stage of embryonic development, and continues in fetal development until birth.
The first sperm cell to successfully penetrate the egg cell donates its genetic material (DNA) to combine with the DNA of the egg cell resulting in a new organism called the zygote.
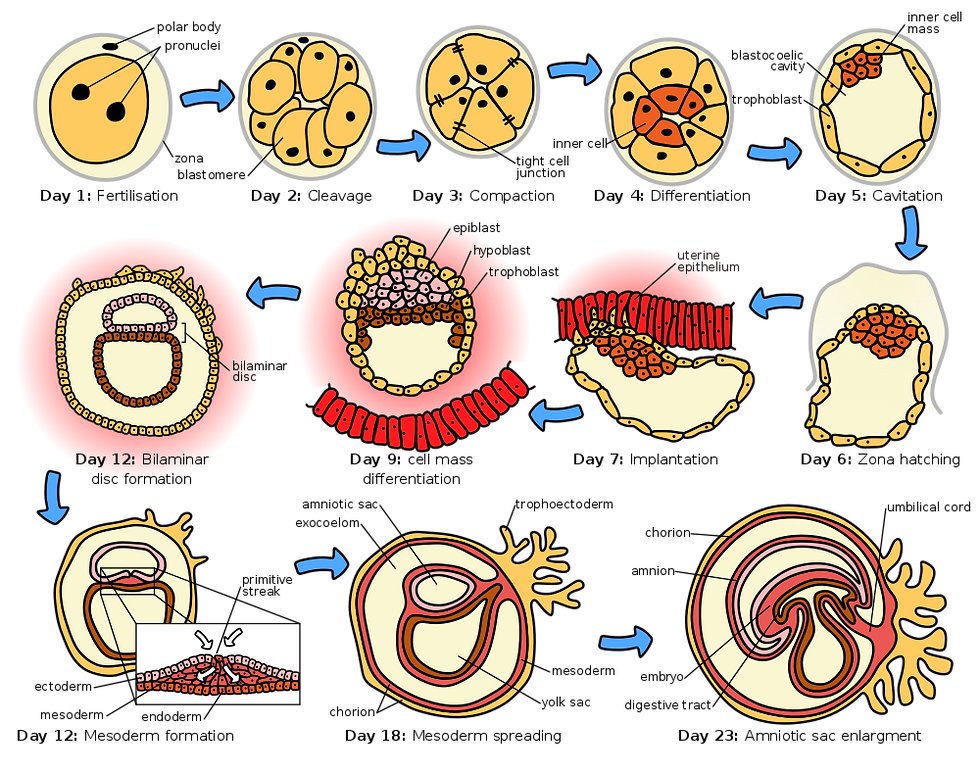
Following fertilization, the embryonic stage of development continues until the end of the 10th week (gestational age) (8th week fertilization age).
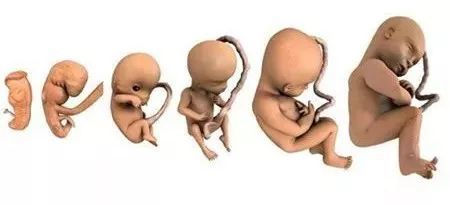
Development of the fetus
Fetal development is the third of the three stages of prenatal development, following from the initial germinal stage (preembryonic stage), and stage of embryonic development. These stages are also referred to in pregnancy as terms or trimesters.[11]
From the 10th week of gestation (8th week of development), the developing organism is called a fetus.
All major structures are already formed in the fetus, but they continue to grow and develop. Since the precursors of all the major organs are created by this time, the fetal period is described both by organ and by a list of changes by weeks of gestational age.
Because the precursors of the organs are now formed, the fetus is not as sensitive to damage from environmental exposure as the embryo was. Instead, toxic exposure often causes physiological abnormalities or minor congenital malformation.
Development of organ systems
Development continues throughout the life of the fetus and through into life after birth. Significant changes occur to many systems in the period after birth as they adapt to life outside the uterus.
Fetal blood
Hematopoiesis first takes place in the yolk sac. The function is transferred to the liver by the 10th week of gestation and to the spleen and bone marrow beyond that. The total blood volume is about 125 ml/kg of fetal body weight near term.
Red blood cells
Megaloblastic red blood cells are produced early in development, which become normoblastic near term. Life span of prenatal RBCs is 80 days. Rh antigen appears at about 40 days of gestation.
White blood cells
The fetus starts producing leukocytes at 2 months gestational age, mainly from the thymus and the spleen. Lymphocytes derived from the thymus are called T lymphocytes (T cells), whereas those derived from bone marrow are called B lymphocytes (B cells). Both of these populations of lymphocytes have short-lived and long-lived groups. Short-lived T cells usually reside in thymus, bone marrow and spleen; whereas long-lived T cells reside in the blood stream. Plasma cells are derived from B cells and their life in fetal blood is 0.5 to 2 days.
Glands
The thyroid is the first gland to develop in the embryo at the 4th week of gestation. Insulin secretion in the fetus starts around the 12th week of gestation.
Factors influencing development
Poverty, mother’s age, drug use, alcohol, tobacco use, infection, nutrition, stress, environmental toxins, low birth weight
The fetus grows and develops in a very complex environment in the body. This system is mainly composed of 3 main parts, which are interdependent and work together to form an organic whole. The baby's earliest home includes the placenta, the umbilical cord and the amniotic fluid, which together provide nutrition, temperature and protection for the growth and development of the fetus until the fetus is fully mature and grows independently from the uterus.
Placenta The placenta is a soft, round or oval-shaped organ that grows as the fetus grows. At 10 weeks gestation, the placenta weighs about 12 grams, and by the time of delivery it weighs about 700 grams.
When the fertilized egg implants in the uterus, the placenta begins to grow, extending many blood vessels into the uterine wall. These blood vessels carry oxygen and nutrients from the mother body's blood to the fetus. Fetal waste is transported back through the placenta into your bloodstream and excreted by the mother.
We used to think that the placenta created a barrier between the fetus and foreign substances. But now know that's not the case. In some cases, the placenta does not completely protect the fetus from harmful substances ingested by the mother, such as alcohol, many drugs, some harmful substances (such as nicotine) and certain vitamins and minerals. This is one of the reasons why pregnant women avoid exposure to many substances at this stage.
The placenta is important for pregnancy and continues to play an important role until delivery. During labor, the uterus begins to contract, the placenta is detached from the uterus, and the fetus is delivered along with it.
Umbilical cord The umbilical cord is the organ that connects the fetus to the placenta and is usually 61 cm long. The umbilical cord is gray, curled, or thick, and contains two arteries that absorb blood and nutrients from the placenta. The veins in the umbilical cord carry oxygen from the blood back to the fetus.
The umbilical cord is occasionally knotted, but it is relatively rare. Doctors believe this is due to fetal movement during the first trimester. The umbilical cord first forms a sleeve. When the fetus drills through the sleeve, a knot is formed. There is no way to prevent the umbilical cord from being knotted.
Amniotic sac The amniotic sac is a sac in the uterine cavity that contains the fetus and the amniotic fluid that surrounds the fetus. In the first trimester, the amniotic fluid comes from the encased placenta and amniotic membrane. In the third trimester, the amniotic fluid is mainly composed of fetal urine and secretions from the lungs.
As the gestational age increases, the amount of amniotic fluid also begins to increase. As labor nears its peak, it begins to slowly decline.
Amniotic fluid can maintain the tension of the amniotic sac, which can ensure the free movement of the fetus and help the development of muscles and joints. Amniotic fluid regulates body temperature, provides cushioning, and ensures that the fetus is not harmed. It also promotes the early maturation of the fetal lungs through the fetus's excretion of fluids.
At 21 weeks of pregnancy, the fetus's digestive system has matured to ensure that the fetus can swallow the amniotic fluid, which helps the fetus to have a mature digestive system function after birth. By the time of labor, the fetus can swallow up to 500 ml of amniotic fluid a day.
Amniotic fluid is an important indicator to reflect whether the fetal development is normal. If a puncture test is performed through the amniotic cavity, the amniotic fluid can be withdrawn from the uterine cavity for study. The amount of amniotic fluid also reflects the health of the fetus. The amount of amniotic fluid in the amniotic sac can be measured using ultrasound technology. Polyhydramnios may indicate a possible fetal spinal or digestive malformation, while oligohydramnios may indicate a fetal bladder or kidney problem.
Presence of Meconium Meconium refers to the indigestible residue present in the digestive system of the fetus after swallowing the amniotic fluid. Meconium is a dark-green or slightly brownish substance that is excreted into the amniotic fluid by the fetus's intestines. This can happen before or during labor.
Fetal distress may occur if meconium is present in the amniotic fluid. If the fetus has meconium in the amniotic fluid before delivery, aspiration into the lungs may cause aspiration pneumonia.
When the water breaks, you can see whether there is meconium in the amniotic fluid. Until then, only amniocentesis can be used to judge. If meconium is found during labor, it can be sucked out with a small straw inserted into the newborn's mouth and throat so that it is not swallowed.
コメント